Precision Medicine
Rapidly scalable, all-inducible neural organoids could facilitate drug screening for neurological diseases
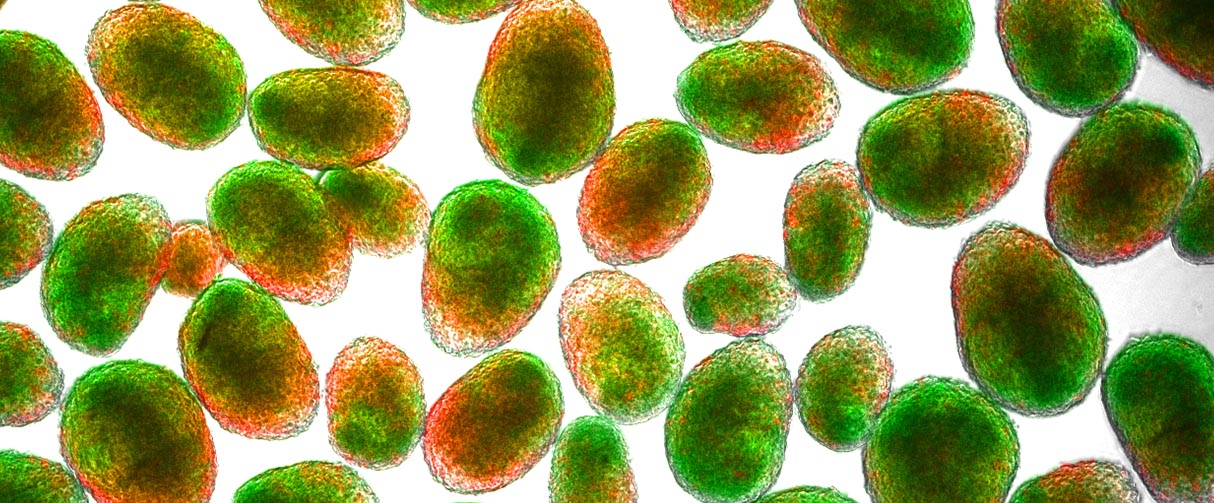
State-of-the-art neural organoids designed by Houston Methodist researchers are scalable, reproducible and allow manipulation of neuron and astrocyte activity
Diseases affecting the brain compromise the function of not just nerve cells but also that of their support staff, the glial cells. In a new study, researchers at Houston Methodist have designed neural organoids, also known as “miniature brains”, to contain both mature neurons and astrocytic glial cells in relative proportions similar to that in the human brain.
Furthermore, they genetically engineered these organoids so that the activity of both cell types can be manipulated independently and on-demand, facilitating the emulation of brain activity during healthy and disease states.
“The ultimate goal is to recapitulate the functionality of the nervous system using organoids, and this study describes a next generation of that technology,” said Robert Krencik, PhD, assistant professor of the Department of Neurosurgery at the Center for Neuroregeneration, and the senior author of the study. “Our new experimental procedure to produce the mature organoids is scalable, reproducible and much faster than previous techniques, taking weeks rather than months.”
These enhanced properties of the neural organoids open doors to multiple applications, including the rapid screening of drugs for neurological diseases, the researchers said.
The new neural organoid technology is published online in the Journal of Cell Biology.
Our new experimental procedure to produce the mature organoids is scalable, easily reproducible and much faster than previous techniques, taking days rather than months.
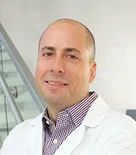
Robert Krencik, PhD
Robert Krencik, PhD
Assistant Professor of Neurosurgery
Houston Methodist
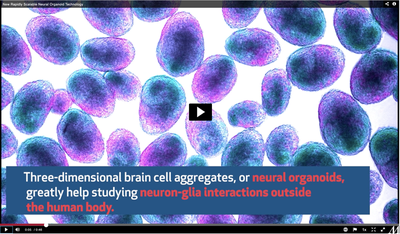
Defined as multicellular, three-dimensional aggregates of cells, organoids are created to simulate the structure and function of organs. These artificially generated mini organs enable scientists to investigate questions that would otherwise require probing of the organ within the body. Also, despite being an in vitro platform, the organoids provide an edge over in vivo research with model organisms since the cellular aggregates for the organoids are derived from human stem cells, thereby retaining key characteristics of human tissue.
Traditionally, brain organoids are slowly developed from human pluripotent stem cells that differentiate into many of the cell types found in the human central nervous system. However, these organoids have certain setbacks: They contain a large number of cell types, including different subtypes of neurons, glial cells and non-neural cells in different states of maturity. Hence, studying the interactions of neurons with any single glial population poses a challenge. Also, neurogenesis and gliogenesis do not happen in tandem, slowing down the process of making mature neural organoids.
“In the brain, synaptic connections between neurons develop and mature after astrocytes are born,” said Krencik. “Currently, using traditional methods, we have to wait several months for the astrocytes to spontaneously generate in the organoids.”
He added that even after the astrocytes appear, the organoids still take extensive time to show brain-like activity that is a hallmark of neural networks.
Addressing these shortcomings, Krencik and his team designed a technique to rapidly generate neural organoids with just neurons and astrocytes. First, instead of neural progenitor cells, they used human pluripotent stem cells to independently create both cell types. To speed up the maturation of astrocytes, they inserted astrocyte-specific transcription factors into the genome of stem cells. In addition, they also included a transgene for a synthetically made receptor into the astrocytes. This genetic manipulation allowed them to activate the astrocytes experimentally with a chemical rather than with neurotransmitters, which would require a release from nerve terminals.
For neurons, on the other hand, they inserted a transgene for channelrhodopsin, a light-sensitive protein that allows positive ions to surge into the neuron upon light activation, causing the cell to depolarize and fire electrical spikes.
“Astrocytes are more responsive to neurotransmitters through their metabotropic receptors, while neurons have the membrane properties needed to fire spikes,” said Krencik. “And so, by using two different technologies to target each cell type, we could selectively activate either neurons or astrocytes.”
The researchers then combined the mature astrocyte and neuron cultures to make spherical organoids and then recorded their electrical activity using a microelectrode array. When they activated the neurons using blue light, they found that they could evoke spikes from these cells to simulate electrical activity of neural networks in the brain.
The effect of stimulating the engineered astrocyte receptor with the chemical clozapine N-oxide (CNO), depended on whether the activation was acute or chronic. When the researchers activated the astrocytes for a few hours, the cells expressed a variety of genes, particularly those that are important for neurons to form synapses. But when chronically activated, the astrocytes transitioned to a detrimental signature with upregulation of neuroinflammatory molecules. However, these adverse reactions could be rescued if growth factors were added to media containing the organoids.
“Overactive astrocytes seem to reduce the viability of the neurons but that could be protected in more optimal conditions,” said Krencik. “So, the external environment likely has an important role, which might have implications for brain stimulation in the clinic, for example, where one has to be careful not to stimulate too much.”
In their next steps, the researchers plan to add more complexity to the organoids by including other cell types and optimizing experimental methods to increase brain wave-like activity. In parallel, they will use their organoids to pursue basic science and translational research questions.
“Our all-inducible organoid system is a useful tool to not just understand interactions between neurons and astrocytes in the healthy brain, but also how these connections are altered by disease,” said Krencik. “An exciting application for this technology is drug discovery. It can be scaled up very quickly to make thousands of organoids at once that can then be used as a high-throughput testing platform for therapeutic drugs for different neurological diseases, including Parkinson’s, Alzheimer’s and cancers of the nervous system.”
Caroline Cvetkovic, Rajan Patel, Arya Shetty, Matthew K. Hogan, Morgan Anderson, Nupur Basu, Samira Aghlara-Fotovat, Srivathsan Ramesh, Debosmita Sardar, Omid Veiseh, Michael Ward, Benjamin Deneen, Philip J. Horner. Assessing Gq GPCR-induced human astrocyte reactivity using bioengineered neural organoids. Journal of Cell Biology. 2022 Feb 09; 221 (4): e202107135. https://doi.org/10.1083/jcb.202107135
This research is supported by grants from the National Institute on Aging of the National Institutes of Health (R21AG064567), the Mission Connect program of The Institute for Rehabilitation and Research Foundation (019-114), The Michael J. Fox Foundation for Parkinson’s Research (17871) and the Cancer Prevention and Research Institute of Texas (RP200655).
Vandana Suresh, PhD, February 2022
Related Articles