Follow us
Copyright 2024. Houston Methodist, Houston, TX. All rights reserved.
Restorative medicine
Novel Vascular Engineering Platforms Are a Boon for Bioengineering
Owing to a dearth of donated organs for transplantation, scientists at Houston Methodist have made a significant breakthrough the field of organ engineering
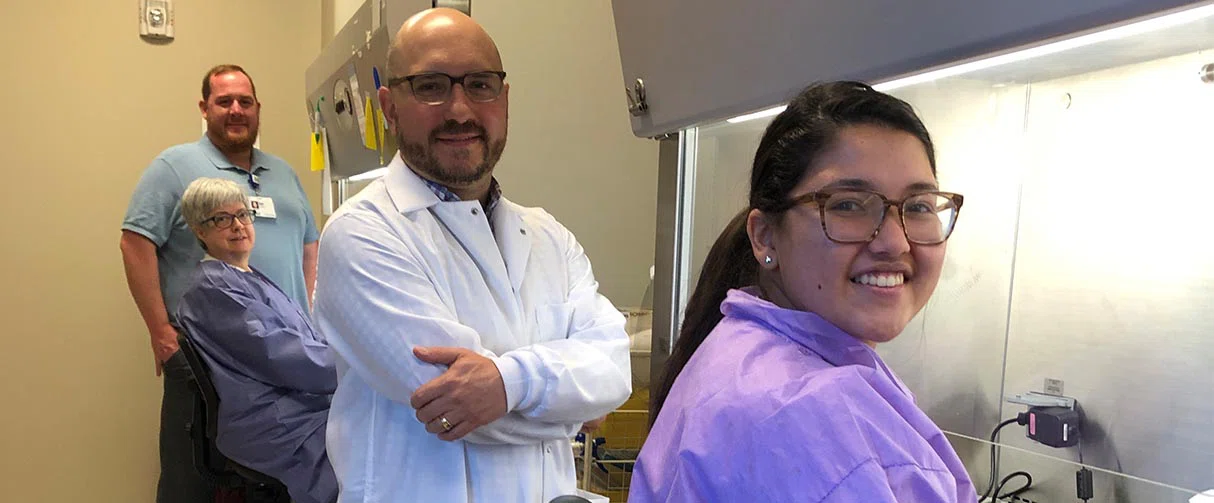
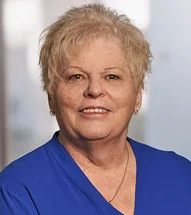
Joan Nichols, PhD
Professor of Immunology in Surgery
As of June 2022, 106,084 people in the US are waiting for a life-saving organ transplant. Every nine minutes, another person is added to the transplant waiting list, and 17 people die each day waiting for a transplant. The dearth of donated organs has inspired scientists to investigate application of bioengineering principles to generate human organs in the lab. Bioengineered organs could potentially not only replace donor organs but provide an unlimited supply. In addition, there would be no immune suppression required, improving success rates of transplant surgeries. The field of bioengineered organs has been advancing at a steady pace, but Houston Methodist’s Joan Nichols, PhD, and her team have made a breakthrough in organ engineering that brings clinical translation one step closer to reality and can also be easily adapted to many other applications.
Bioengineering of a whole organ requires a scaffold upon which the cells can grow, develop and function. In a complex organism this role is filled by the extracellular matrix. For bioengineering, a biological scaffold is generated by decellularization of naturally occurring human or animal tissues and organs. Removing the cells removes the allogeneic and xenogeneic cellular antigens thus eliminating the immune response that would lead to organ rejection.
In 2018, Nichols’ team published a paper in Science Translational Medicine revealing their success with a bioengineered lung transplanted in a clinically relevant porcine model. And while their model functioned to produce systemic vascular circulation, it was not suitable for development of functional pulmonary circulation with bioengineered pulmonary artery and vein. Nichols attributed the lack of vascular tissue development to limited dispersal of the growth factor needed for vascular tissue development —vascular endothelial growth factor (VEGF) — and other additives in the whole organ scaffold, combined with the inability of the system to retain the growth factor delivery suspension within the scaffold framework. VEGF is critical in vascular development and has been shown to support development of vascular tissue in bioengineering, but it needs to make it to the scaffold and remain there to do the job.
Generating vascular tissue with microvasculature networks capable of perfusion at physiological pressures without leaking is perhaps the most common challenge in organ and tissue bioengineering. The biomimetic vascular models available have been unable to model vascular cell responses and lacked the appropriate vascular culture platforms focused on 3D whole vessel or whole lung vascular bioengineering. While microfluidic-supported platforms are the standard for investigating endothelial cell culture methods and vascular tissue responses, they are unable to accommodate evaluation of whole vessel or whole organ culture.
These vascular models are designed for growth factor delivery that enhances angiogenesis. Why would you want to enhance angiogenesis? Angiogenesis drives vascularization in everything from wound healing and tissue regeneration to transplanted organs.”
Joan Nichols, PhD
Professor of Immunology in Surgery
Houston Methodist
So, Nichols’ group did what they do best and developed what they needed to advance their work: novel in vitro biomimetic vascular models with appropriate culture- or microfluidic-supported vascular platforms to assess vascular cell responses to growth factors and factor delivery vehicles. These platforms include flattened pieces of vascular scaffold with standard plate culture; microfluidic system-supported whole-vessel scaffolds and whole acellular lung scaffolds. The whole vessel and whole lung platforms are microfluidic-supported culture chambers that mimic native tissue function: they allow medium exchange and provide nutrient and oxygen circulation and waste removal from the culture micro-environment.
In the study published last summer in Nanomedicine: Nanotechnology, Biology and Medicine, Nichols’ team used these platforms to investigate delivery of VEGF via microparticle (MP) or hydrogel vehicles to the scaffolds and the effects of VEGF on vascular cell attachment and subsequent tissue formation in in vitro bioreactor culture. Their results are exciting for several reasons.
IVIS imaging of their system shows that VEGF-MP size and shape allowed retention in the mesh-like collagen/elastin network comprising the acellular whole-lung scaffold but did not impede MP dispersal through its vascular tree. Importantly, they detected no evidence of VEGF-MPs or degraded VEGF-MPs outside of the vascular scaffold and particles were distributed throughout whole left lung scaffolds including in the small capillary regions. Ten days post culture, VEGF could be detected in degrading VEGF-MPs. The effects of VEGF on the scaffolds: endothelial cell attachment and microvascular and vascular tissue formation, were found only in the MP-targeted vascular regions. In addition, they found that a 50/50 mixture of 30 and 60 nm pore-sized silicon wafer MPs was superior to the hydrogel for delivering VEGF. While hydrogels are thermosensitive and cannot provide slow, controlled release of growth factors, MPs with the appropriate pore size provide temporospatial release of factors to facilitate both protein loading and load release. Furthermore, they demonstrated that MPs could fit into even the smallest vascular regions and in whole organ scaffolds. These results show that MPs are more effective than hydrogel at delivery of bioactive factors in acellular whole lung scaffolds for facilitation of vascular tissue bioengineering.
The success of silicon MPs for factor delivery is especially exciting for the future of vascular and organ bioengineering because standardization of pharma-grade MPs can be achieved by leveraging well-characterized large-scale manufacturing techniques borrowed from the semiconductor industry. These techniques have the scalability to support research & development studies, clinical trials and, ultimately, clinical commercial use.
Their results also demonstrate that the newly developed platforms provide the modeling capabilities researchers in this field need to keep advancing their work. But these platforms not only signal important advancement for whole organ and vascular bioengineering, they can be adapted for use in other studies. They allow assessment of growth factors (alone or in combination) and delivery systems and can be easily modified to evaluate, for example, therapeutics for clinical vascular graft production, vascular regeneration, vascular health and more.
Currently, Nichols and her team are working on using these platforms to model select agent and SARS-CoV-2 infections and test candidate countermeasures.
Lissenya B. Argueta, Jean A. Niles, Jason Sakamoto, Xuewu Liu, Stephanie P. Vega, Luba Frank, Marco Paessler, Joaquin Cortiella, Joan E. Nichols https://www.sciencedirect.com/science/article/abs/pii/S1549963421000629?via%3Dihub
Heather Lander, PhD, June 2022
Related Articles
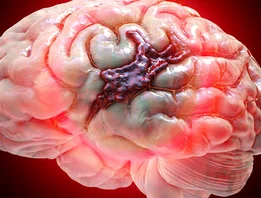
RESTORATIVE MEDICINE
Improved Translational Models to Probe Brain Hemorrhage
A battery of validated rodent behavioral tests now facilitates investigating the underpinnings of neurocognitive deficits after a subarachnoid hemorrhage.
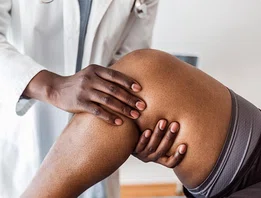
RESTORATIVE MEDICINE
Restoring Muscle Strength Gets a Helping Hand from Blood Flow Restriction Training
Muscle strengthening exercises are an essential part of recovery after certain injuries or surgeries, but they can be prohibitive for patients experiencing pain. As a workaround solution, combining blood flow restriction and low-intensity strength training is an equally effective way to build muscle endurance and power.